Research - (2022) Volume 10, Issue 12
The effect of clinical sandblasting with different powders on the surface roughness of cores for metal-ceramic crowns and their fracture resistance after the addition of repair material
Mohammed Thamir Yassin1* and Shatha Abdullah Salih2
*Correspondence: Mohammed Thamir Yassin, School of Dentistry, University of Sulaimani, Iraq, Email:
Abstract
Background/ purpose: The most frequently encountered issue with metal-ceramic restorations is the fracture of veneering porcelain. This in vitro study aims to evaluate the effect of clinical sandblasting with 50 μm aluminum oxide and 30 μm silica-coated particles on the surface roughness of metal cores and the subsequent effect on their fracture resistance after the addition of specific adhesive and packable composite as a repair material. Materials and Methods: Metal cores (n=21) were digitally designed and three-dimensionally printed by selective laser melting technique by ProX 100 3D printer (3D Systems, Inc). These cores were randomly divided into three groups: Group A: n=8, sandblasted with 50 μm aluminum oxide, and veneered with packable Z350 composite. Group B: n=8, sandblasted with 30 μm silica-coated particles and veneered with packable Z350 composite. Group C: control group (n=5), sandblasted in the laboratory with 250 μm aluminum oxide and veneered with porcelain (Vintage PRO–SHOFU0). All the specimens were tested for surface roughness by the TAYLOR-HOBSON profilometer. After adding the veneering material, all the specimens were subjected to a fracture resistance test through a universal testing machine. Results: One-way ANOVA test showed a significantly higher difference for the specimens sandblasted in the laboratory using 250 μm aluminum oxide. Fracture resistance values showed no significant difference between groups A and B. Conclusion: Groups A and B showed no significant difference in surface roughness, but their fracture resistance values were above the acceptable clinical limit.
Keywords
Intra-oral repair, Sandblasting, Silica, Surface roughness
Introduction
Dental esthetics can be defined as the science and art of enhancing the aesthetics and function of the teeth, oral cavity, and facial symmetry via the application of certain knowledge and methods [1]. In the late 1700s, ceramics were introduced as restorative materials in dentistry, taking advantage of their ability to mimic the form and color of natural teeth [2]. There are three basic types of materials that are used for indirect dental restorations, and these materials include metal alloys (both all-metal and metal-ceramic), ceramics, and resinbased composites [3]. Porcelain fused to metal (PFM) restorations have the benefit of combining clinical durability with acceptable cosmetic results. However, there are post-insertion complications with metalceramic crowns and fixed partial dentures [4]. Biological or technical complications are possible. One of the most common issues with metal-ceramic restorations is the chipping of the veneering material [5]. It may not be the most practical alternative to replace the entire restoration because it is time-consuming, expensive, and there is a risk of traumatizing the abutment [6]. On the other hand, repairing the broken porcelain intraorally is quite simple and offers a cost- and time-effective alternative to the patient and the dentist, ensuring that both function and esthetics are restored [7].
The computer-aided design/computer-aided manufacturing (CAD/CAM) laser melting is an additional fabrication process for metal copings of metal-ceramic crowns [8]. This technique does not have the limitations of the conventional waxing and lost-wax technique. Selective laser melting (SLM) uses high-temperature laser beams for selective heating of the metal framework, which is in the form of powder, and uses the CAD data obtained from designing the framework for this purpose [9].
Sandblasting (SA), grinding, acid or heat etching, or combinations thereof, are some of the most frequent surface roughening techniques [10,11]. One of the most popular surface treatment methods, airborne-particle abrasion (sandblasting), causes micro undercuts, increases the substrate’s surface roughness, and enhances micro-mechanical bonding agent retention [12]. There are a variety of particle sizes (ranging from 25 μm to 250 μm) and compositions (such as ordinary alumina or silica-coated particles) available for abrasion by airborne particles [13,14]. In the existing literature, there are little data regarding the effect of clinical sandblasting on metal cores fabricated by the SLM technique; hence this study aims to investigate the effect of clinical sandblasting with 50 μm aluminum oxide and 30 μm silica-coated particles on the surface roughness of metal cores and the subsequent effect on their fracture resistance after the addition of repair material.
Materials and Methods
Tooth Preparation: Lower right first molar was digitally designed using Exocad software (Exocad 3.0 Galway) according to the dimensions of a natural tooth as documented by Chatterjee Kabita, et al. [15]. Using the same software, a digital preparation was performed with the following guidelines: Occlusal reduction: 1.5 mm at the center and 2 mm at the cusp tip with 45-degree beveling. The other surfaces were reduced with a taper of 6 degrees. Finishing line: 1.3 mm all around shoulder with internal roundation (Figure 1A). 21 definitive Poly Methyl Methacrylate (PMMA) (DETAX, Germany) casts were digitally printed using Arum 5x-300 Pro milling machine corresponding to the previously mentioned parameters. Each cast was attached to a 3D-printed, cylindrical acrylic block (MAZIC, VERICOM CO., LTD.) with a height of 20 mm, and a diameter of 16 mm (Figure 1B). These are considered the abutments to receive the cores and the crowns.
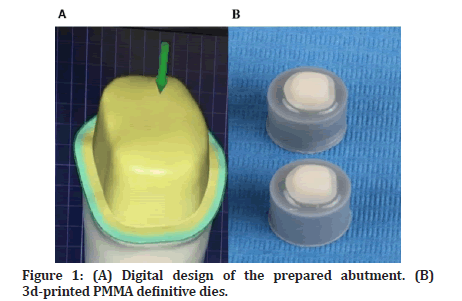
Figure 1: (A) Digital design of the prepared abutment. (B) 3d-printed PMMA definitive dies.
Fabrication of Metal Cores and Metal-ceramic Crowns: Using Exocad 3.0 Galway software, metal cores (n=21) were designed with a thickness of 0.5 mm (Figure 2A) according to manufacturer instructions and threedimensionally printed by Selective Laser Melting Technique with Cobalt-Chrome metal powder (3D Systems, Inc) by ProX 100 3D printer (3D Systems, Inc) (Figure 2B) with an accuracy of 30 microns per each slice, followed by heating up to 800 °C for 1 hour using programat p310 furnace (Ivoclar Vivadent AG).. These cores were randomly divided into three groups: group A n=8 (sandblasted clinically with 50 μm aluminum oxide), group B n=8 (sandblasted clinically with 30 μm silica-coated particles), and group C n=5 (control group: sandblasted in the laboratory with 250 μm aluminum oxide). Specimens of the control group were veneered with porcelain according to manufacturer instructions (Vintage PRO - SHOFU Dental GmbH).
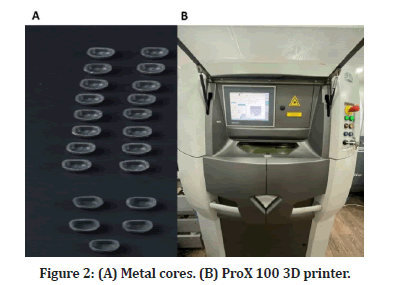
Figure 2: (A) Metal cores. (B) ProX 100 3D printer.
Sandblasting and Surface Roughness Test: Specimens of the control group were sandblasted in the laboratory using 250 μm aluminum oxide (Korox; BEGO Medical) at 2 bar pressure from 50 mm distance as a setting for laboratory work. The specimens from group A were clinically sandblasted with 50 μm aluminum oxide (Dentify GmbH, Germany), while the specimens from group B were clinically sandblasted with 30 μm silicacoated particles (3M™ Cojet™ Sand). Clinical sandblasting parameters were set as follows: 2.5 bar pressure from 10 mm distance for 10 seconds by using a clinical sandblasting device (AquaCare - Velopex International). The sandblaster handle was attached to a customized dental surveyor (Ney Surveyor, Ney Dental, Bloomfield, CT, USA) to allow for standard movement during the process of sandblasting (Figure 3A and 3B). All the samples were tested for surface roughness using the TAYLOR-HOBSON profilometer (Figure 3C). Specimens of the control group were tested twice, before and after sandblasting. Three readings for each specimen were recorded at a 1 mm distance between each line, one in the center and the other two at a 1 mm distance above and below, and the mean value was calculated.
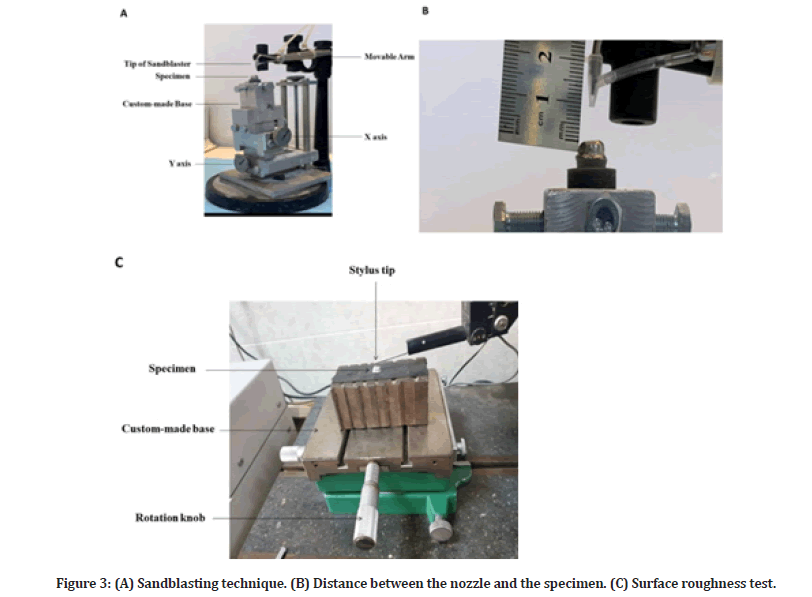
Figure 3: (A) Sandblasting technique. (B) Distance between the nozzle and the specimen. (C) Surface roughness test.
Cementation
All the specimens were cemented using dual-cure selfadhesive resin cement TheraCem (Bisco, Schaumburg, USA) following manufacturer instructions. A 5-kg weight was used to keep the samples in place during the primary cement setting to ensure uniform seating pressure.
Application of Repair Material and Fracture Load Test: Composite build-up was performed on all the specimens from groups A and B using light-cure resin composite (3M Filtek Z350 XT). A transparent mold with a thickness of approximately 1 mm (Figure 4A) was fabricated by using clear polyvinyl siloxane (EXACLEAR; GC Corp) on a randomly chosen specimen from the control group to control the thickness of the composite material. A layer of veneer wax (Renfert GmbH) was added beneath the finishing line of the definitive die to block the undercut and control the fit of the mold (Figure 4B). Before the addition of veneering composite, the porcelain repair kit (Intraoral Repair Kit, Bisco Inc., Schaumburg, IL) was used according to the manufacturer’s instructions; one coat of Z-Prime Plus was applied and dried with an air syringe for 3-5 seconds. A thin layer of porcelain bonding resin was applied and spread evenly on the surface, then air-thinned for 3-5 seconds. For each specimen, the mold was loaded with two capsules of (3M Filtek Z350 XT) and secured over the specimen; excess composite was removed with a micro brush and then light cured for 20 seconds for each occlusal, buccal, lingual, mesial, and distal surface using light curing pen (Eighteeth, Changzhou, China) at an intensity of 1000 mW/cm² from a distance of 1-2 mm. After the removal of the transparent mold (Figure 4C), each surface was light-cured for another 20 seconds. The specimens were then kept in 37 °C distilled water for one week. All the specimens were then subjected to 1000 rounds of thermal cycling between 5±2 ºC and 55±2 ºC for 30 seconds in each bath and 5-second interval between the baths. All the specimens were then loaded by a universal testing machine (TERCO MT 3037 Terco I&S AB, Sweden). Each specimen was secured in a custom-made metallic base, and the pressure was applied through a vertically movable rod with a semi-spherical head of 6 mm in diameter (Figure 4D), with a cross speed of 1 mm/ min. The loading piston was positioned at the center of the occlusal surface (Figure 4E). In order to make sure that the position was correct, it was checked by three examiners.
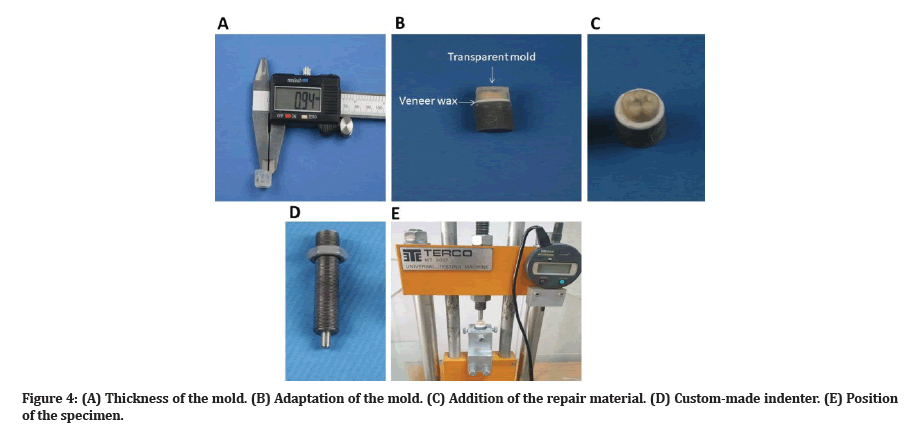
Figure 4: (A) Thickness of the mold. (B) Adaptation of the mold. (C) Addition of the repair material. (D) Custom-made indenter. (E) Position of the specimen.
Statistical analysis
Following a one-way analysis of variance (ANOVA), the normality and homogeneity of variance were tested using Shapiro-Wilk and Levene's tests, respectively. Paired t-tests were computed for groups measured twice, and independent two-sample t-tests were used to compare two groups. The Bonferroni test was used to detect multiple comparisons among the experimental groups mentioned above. SPSS version 25 was used to run all the statistical tests. A significant difference was set at P<0.05.
Results
Surface Roughness Table 1 shows descriptive statistics of surface roughness measurements where the data is present as mean ± SD. No statistically significant difference was found between groups C before sandblasting, A, and B.
N | Mean ± SD | Minimum | Maximum | Test Value (P-Value) | ||
---|---|---|---|---|---|---|
Paired Test | C - Before SA | 5 | 1.553 ± 0.112 | 1.42 | 1.68 | -11.018 (0.000) |
C - After SA | 5 | 2.280 ± 0.100 | 2.12 | 2.35 | ||
One-Way ANOVA | C - Before SA | 5 | 1.553 ± 0.112 | 1.42 | 1.68 | 0.151 (0.861) |
A - After SA | 8 | 1.588 ± 0.203 | 1.4 | 1.9 | ||
B - After SA | 8 | 1.535 ± 0.215 | 1.2 | 1.833 |
Table 1:Statistical Paired T-Test and One-Way ANOVA test for surface roughness values (μm).
Fracture resistance
Table 2 shows descriptive statistics of fracture resistance measurements where the data is present as mean ± SD. Although B showed higher values of fracture resistance than A, no statistically significant result was concluded with a p-value= 1 as shown in table 3.
N | Mean ± SD | Std Error | Minimum | Maximum | ||
---|---|---|---|---|---|---|
Fracture Resistance/N | C | 5 | 2310.000 ± 527.210 | 235.775 | 1730 | 2910 |
A | 8 | 1311.250 ± 301.777 | 106.694 | 930 | 1770 | |
B | 8 | 1328.750 ± 299.306 | 105.821 | 1110 | 2000 |
Table 2: Descriptive statistical result for fracture resistance measure per group (N).
Mean Difference (I-J) | Std. Error | Sig. | 95% Confidence Interval | |||
---|---|---|---|---|---|---|
Lower Bound | Upper Bound | |||||
C (PFM) | A | 998.750* | 207.139 | 0 | 452.079 | 1545.421 |
B | 981.250* | 207.139 | 0 | 434.579 | 1527.921 | |
A | B | -17.5 | 181.673 | 1 | -496.962 | 461.962 |
Table 3: Bonferroni pairwise comparison test result.
Effect of Surface Roughness on Fracture Resistance: linear regression modeling was built to highlight the variability between surface roughness and fracture resistance. The scatter plot displays that there was a positive linear relationship between surface roughness and fracture resistance, as shown in Figures 5A and Figure 5B.
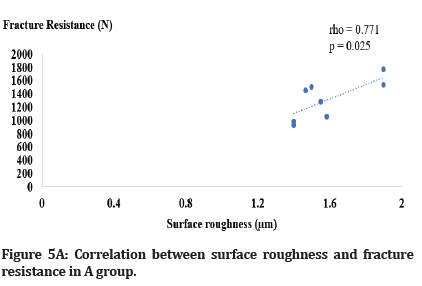
Figure 5A: Correlation between surface roughness and fracture resistance in A group.
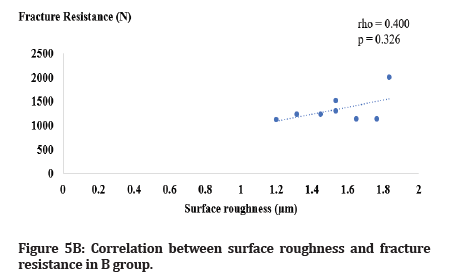
Figure 5B:Correlation between surface roughness and fracture resistance in B group.
Discussion
The gold standard in posterior tooth indirect restorations is a metal-ceramic fixed partial prosthesis [16]. When it comes to metal-ceramic restorations, the chipping of the veneering ceramic is considered to be one of the most common issues that can arise [17]. In the present study, preparation parameters were selected to provide sufficient thickness for both metal cores and veneering material according to manufacturer instructions. Since the modulus of elasticity of poly methyl methacrylate is comparable to that of human dentin [18], it was decided that this would be the material of choice for the production of definitive dies.
The selective laser melting technique (SLMT) can produce Co-Cr restorations with qualities comparable to or superior to casting and CAD/CAM milling at a fraction of the time and cost [19]. In addition to producing restorations with more uniform quality, SLMT standardizes the process of shaping restorations, uses less personnel, and has the potential to produce greater accuracy because it eliminates multiple procedural steps like waxing up, flasking, and casting [20]. It is worth mentioning that the surface roughness of Co- Cr specimens is affected by manufacturing processes. After Additive Manufacturing (AM) of Co-Cr specimens, a post-processing heat treatment of Co-Cr metal alloys is necessary to alleviate internal tensions induced by temperature gradients during the process. The surface roughness of SLM specimens is determined by their layer-by-layer configuration [21].
An airborne-particle abrasion method (most typically sandblasting using alumina particles) is frequently used to remove contaminants, roughen the substrate surface, and alter the wettability and energy of the substrate [22]. In the present study, the sandblasting parameters, including the size of particles, distance, pressure, and duration, have been selected based on the previous study performed by Okada et al. [14], as this combination showed the most favorable outcome regarding flexural strength. In terms of sandblasting effect, the results of the present study showed that there was no significant change in the surface roughness of the metal cores after clinical sandblasting with either 50 μm aluminum oxide or 30 μm silica-coated particles. This could be explained by the rough nature of the metal surface fabricated by the selective laser melting technique, which is supported by the finding of Alqahtan, et al. [23] as there were increased porosities and micro-irregularities evident on the untreated metal surface. The results of the present study also go in line with the finding of Revilla-León et al. [24] as it showed that those surface roughness readings of Co-Cr specimens produced by milling technique (subtractive) which were sandblasted using 100 μm aluminum oxide were significantly lower than untreated specimens produced by SLMT. Sandblasting with silica-coated particles showed the least roughness value; this could be ascribed to the chemical interaction between the silica particles and the metal substrate, which maintains the silica particles lodged in the metal substrate's surface; thus, filling the micro-porositites [25]. Laboratory sandblasting with 250 μm aluminum oxide showed significantly higher roughness values compared to the other groups; this could be attributed to the larger particle size of aluminum oxide powder [26].
The metal cores for both groups A and B were treated with a specific repairing kit which provides the basis for chemical bonding with the metal oxide generated on base metal alloys. This is because it contains 10-methacryloyloxydecyl dihydrogen phosphate (MDP), which may increase the binding strength to base metal alloys, as stated by Sadighpour, et al. [27]. A vinyl group on the other end of the molecule aids polymerization with the resin matrix's unsaturated carbon bonds. An ester chain with ten carbons serves as a spacer between these two active groups [28].
Regarding fracture resistance, the results of the present study show significantly higher values for the control group; this could be explained by the difference in the processing technique and veneering material [29]. Groups A and B showed no significant differences with slightly higher values in groups B; this could be attributed to the fact that sandblasting with silica coating embeds silica particles in the surface of the substrate, so producing a physically and chemically active outer surface layer (oxide layer), which promotes a chemical adhesion with the phosphate monomer (MDP), resulting in a stronger bonding [30]. It is worth mentioning that all the specimens exhibited higher values than the average bite force in the first molar region, as documented by Khan, et al. [31].
Conclusion
The following conclusions can be drawn from this study:
Clinical sandblasting of metal cores fabricated by SLMT using different powders had no effect on the surface roughness.
In terms of fracture resistance, all the specimens showed values above the acceptable clinical limit.
Conflicts of Interest
The authors have no conflicts of interest relevant to this article.
References
- Goldstein RE, Patzer G. Concepts of dental esthetics. In: Goldstein RE, Chu SJ, Lee EA, et al. Ronald E goldstein’s esthetics in dentistry. John Wiley and Sons 2018; 1–22.
- Naji GAH, Omar RA, Yahya R. An overview of the development and strengthening of all-ceramic dental materials. Biomed Pharmacol J 2018; 11:1553–1563.
- Butt K, Thanabalan N, Ayub K, et al. Demystifying modern dental ceramics. Primary Dent J 2019; 8:28–33.
- Donovan TE, Alraheam IA, Sulaiman TA. An evidence-based evaluation of contemporary dental ceramics. Dent Update 2018; 45:541–546.
- Shirani F, Kianipour A, Rahbar M. The effect of mechanical and chemical surface preparation methods on the bond strength in repairing the surface of metal-ceramic crowns with composite resin: A systematic review and meta-analysis. Maedica 2020; 15:206.
- Aslam A, Hassan S, Nayyer M, et al. Intraoral repair protocols for fractured metal-ceramic restorations-Literature review. South African Dent J 2018; 73:35–41.
- Yadav JS, Dabas N, Bhargava A, et al. Comparing two intraoral porcelain repair systems for shear bond strength in repaired cohesive and adhesive fractures, for porcelain-fused-to-metal restorations: An in vitro study. J Indian Prosthodont Soc 2019; 19:362.
- Liu W, Qing H, Pei X, et al. Internal adaptation of cobalt-chromium posts fabricated by selective laser melting technology. J Prosthet Dent 2019; 121:455–460.
- Gholamrezaei K, Vafaee F, Afkari BF, et al. Fit of cobalt-chromium copings fabricated by the selective laser melting technology and casting method: A comparative evaluation using a profilometer. Dent Res J 2020; 17:200.
- He M, Zhang Z, Zheng D, et al. Effect of sandblasting on surface roughness of zirconia-based ceramics and shear bond strength of veneering porcelain. Dent Materials J 2014;
- Bernevig-Sava M, Stamate C, Lohan N, et al. Considerations on the surface roughness of SLM processed metal parts and the effects of subsequent sandblasting. In IOP Publishing 2019; 572:012071.
- Raeisosadat F, Ghoveizi R, Eskandarion S, et al. Influence of different surface treatments on the shear bond strength of resin cement to base metal alloys. J Lasers Med Sc 2020; 11:45.
- Fonseca RG, de Oliveira Abi-Rached F, Reis JM dos SN, et al. Effect of particle size on the flexural strength and phase transformation of an airborne-particle abraded yttria-stabilized tetragonal zirconia polycrystal ceramic. J Prosthet Dent 2013; 110:510–514.
- Okada M, Taketa H, Torii Y, et al. Optimal sandblasting conditions for conventional-type yttria-stabilized tetragonal zirconia polycrystals. Dent Mater 2019; 35:169–175.
- https://www.jaypeebrothers.com/pgDetails.aspx?cat=s&book_id=9789390595969
- Ispas A, Iosif L, Popa D, et al. Comparative assessment of the functional parameters for metal-ceramic and all-ceramic teeth restorations in prosthetic dentistry: A literature review. Biology 2022; 11:556.
- Pjetursson BE, Valente NA, Strasding M, et al. A systematic review of the survival and complication rates of zirconia‐ceramic and metal‐ceramic single crowns. Clin Oral Implants Res 2018; 29:199–214.
- Pallis K, Griggs JA, Woody RD, et al. Fracture resistance of three all-ceramic restorative systems for posterior applications. J Prosthet Dent 2004; 91:561–569.
- Lu Y, Lin W, Xie M, et al. Examining Cu content contribution to changes in oxide layer formed on selective-laser-melted CoCrW alloys. Applied Surface Sci 2019; 464:262–272.
- Taftali M, Turalioğlu K, Yetim AF, et al. Improvements in the static/dynamic strength of porcelain fused to metal dental crowns with surface protrusions produced by selective laser melting. J Manuf Process 2021; 65:112–118.
- Al Jabbari Y, Koutsoukis T, Barmpagadaki X, et al. Metallurgical and interfacial characterization of PFM Co–Cr dental alloys fabricated via casting, milling or selective laser melting. Dent Mater 2014; 30:e79–88.
- Aurelio IL, Marchionatti AME, Montagner AF, et al. Does air particle abrasion affect the flexural strength and phase transformation of Y-TZP? A systematic review and meta-analysis. Dent Mater 2016; 32:827–845.
- Alqahtani AS, AlFadda AM, Eldesouky M, et al. Comparison of marginal integrity and surface roughness of selective laser melting, CAD-CAM and digital light processing manufactured Co-Cr alloy copings. Appl Sci 2021; 11:8328.
- Revilla-León M, Husain NAH, Methani MM, et al. Chemical composition, surface roughness, and ceramic bond strength of additively manufactured cobalt-chromium dental alloys. J Prosthet Dent 2021; 125:825–831.
- Castillo‐Oyagüe R, Osorio R, Osorio E, et al. The effect of surface treatments on the microroughness of laser‐sintered and vacuum‐cast base metal alloys for dental prosthetic frameworks. Microsc Res Tech 2012; 75:1206–1212.
- Baciu ER, Cimpoeșu R, Vițalariu A, et al. Surface analysis of 3D (SLM) Co–Cr–W dental metallic materials. Appl Sci 2020; 11:255.
- Sadighpour L, Mostafavi AS, Pirmoradian M, et al. Comparison of bond strength in the different class of resin cements to cast and CAD/CAM Co-Cr alloys. Int J Dent 2021; 2021.
- Chen Y, Lu Z, Qian M, et al. Chemical affinity of 10-methacryloyloxydecyl dihydrogen phosphate to dental zirconia: Effects of molecular structure and solvents. Dent Mater 2017; 33:e415–e427.
- Alsadon O, Patrick D, Johnson A, et al. Fracture resistance of zirconia-composite veneered crowns in comparison with zirconia-porcelain crowns. Dent Mater J 2017; 36:289–295.
- Elsantawi AM, Saad D, Shebl A. Evaluation of the shear bond strength of two types of adhesive resin cements to zirconia after surface treatment using silica coating. Dent Sci Updates 2020; 1:23–30.
- Khan SIR, Rao D, Ramachandran A, et al. Comparison of bite force on the dominant and nondominant sides of patients with habitual unilateral chewing: A pilot study. Gen Dent 2020; 68:60–63.
Indexed at, Google Scholar, Cross Ref
Indexed at, Google Scholar, Cross Ref
Indexed at, Google Scholar, Cross Ref
Indexed at, Google Scholar, Cross Ref
Indexed at, Google Scholar, Cross Ref
Indexed at, Google Scholar, Cross Ref
Indexed at, Google Scholar, Cross Ref
Indexed at, Google Scholar, Cross Ref
Indexed at, Google Scholar, Cross Ref
Indexed at, Google Scholar, Cross Ref
Indexed at, Google Scholar, Cross Ref
Indexed at, Google Scholar, Cross Ref
Indexed at, Google Scholar, Cross Ref
Indexed at, Google Scholar, Cross Ref
Indexed at, Google Scholar, Cross Ref
Indexed at, Google Scholar, Cross Ref
Indexed at, Google Scholar, Cross Ref
Indexed at, Google Scholar, Cross Ref
Indexed at, Google Scholar, Cross Ref
Indexed at, Google Scholar, Cross Ref
Indexed at, Google Scholar, Cross Ref
Indexed at, Google Scholar, Cross Ref
Indexed at, Google Scholar, Cross Ref
Indexed at, Google Scholar, Cross Ref
Indexed at, Google Scholar, Cross Ref
Indexed at, Google Scholar, Cross Ref
Indexed at, Google Scholar, Cross Ref
Author Info
Mohammed Thamir Yassin1* and Shatha Abdullah Salih2
1School of Dentistry, University of Sulaimani, Iraq2Department of Conservative Dentistry, College of Dentistry, Hawler Medical University, Iraq
Citation: Mohammed Thamir Yassin, Shatha Abdullah Salih, The Effect of Clinical Sandblasting with Different Powders on the Surface Roughness of Cores for Metal-Ceramic Crowns and their Fracture Resistance after the Addition of Repair Material, J Res Med Dent Sci, 2022, 10 (12): 111-117.
Received: 22-Nov-2022, Manuscript No. jrmds-22-78079; , Pre QC No. jrmds-22-78079(PQ); Editor assigned: 24-Nov-2022, Pre QC No. jrmds-22-78079(PQ); Reviewed: 08-Dec-2022, QC No. jrmds-22-78079(Q); Revised: 12-Dec-2022, Manuscript No. jrmds-22-78079(R); Published: 19-Dec-2022