Research - (2022) Volume 10, Issue 8
Microtensile vs. Flexural Bond Strength for Bond Strength Assessment
Annan Ahmed Elkassaby1*, Mohamed M Kandil1,2 and Ghada Atef Alian1
*Correspondence: Annan Ahmed Elkassaby, Department of Dental Biomaterials, Dentistry, Ain Shams University, Egypt, Email:
Abstract
Introduction: Bond strength tests indicate the performance of different substrates bonded in the oral cavity. Purpose: To evaluate the reliability of the flexural bond strength test as a valid substitute for the microtensile bond strength test. Materials and Methods: Two resin matrix ceramic material (RMC) blocks (Vita Enamic and Lava Ultimate) were subjected to 4 surface treatments: Diamond bur roughening (DBR), DBR + silane application, Hydrofluoric (HF) acid etching (9.5%) and HF acid etching (9.5%) + silane application. The universal bond adhesive was applied following the surface treatments followed by the incremental packing of composite resin. RMC/composite blocks for each group were sectioned by using low-speed cutting saw to produce beam-shaped specimens. These specimens were tested with a universal testing machine for microtensile and flexural bond strength. Data were analyzed using ANOVA, independent t-test, Chi-square test, and Weibull analysis. Results: Lava Ultimate showed significantly higher bond strength values than Vita Enamic. Lava Ultimate showed that DBR + saline application surface treatment had significantly high bond strength values. Vita Enamic showed that DBR + saline application and HF acid etching + saline application had the highest bond strength values. According to Weibull's analysis, the flexural bond strength test showed a higher consistency than the microtensile bond strength test. Conclusion: The flexural bond strength test is a reliable, easy alternative to the highly technique-sensitive microtensile bond strength test.
Keywords
Polymer infiltrated ceramic network, Resin nanoceramics, 4-point bending bond strength
Introduction
Bond strength testing was usually employed to evaluate the performance of dental restorations and adhesives to different substrates, as it reflects the clinical performance and longevity of dental restorations in the oral cavity. Bond strength could be determined by dividing the maximum load to fracture a bonded specimen by the actual cross-sectional area of the bonded interface [1,2]. The ideal bond-strength testing methodology should be comparatively easy to perform. i.e., low technique sensitivity, reasonably fast, and must be reproducible in most research laboratories [3,4].
Various testing methodologies were conventionally employed for bond strength testing, including tensile bond strength, shear bond strength, and push out tests [1,4]. Those testing methods could be performed on the macro and micro-scales. Although macro testing methods were usually preferred due to their simplicity and ease of specimen preparation [1,5], micro testing generally gives higher and more consistent bond strength results due to the presence of fewer and smaller defects/flaws at the bonded interface [6]. Of these conventional tests, the microtensile bond strength (μTBS) test received the most attention due to the improved stress distribution at the adhesive interface, thus preventing cohesive fracture in the substrate [4]. Good economic usage of substrates as numerous specimens can be acquired from one tooth, enabling more creative experiment set-ups and properly managed substrate variables [4].
Nevertheless, it showed inconsistent results among different studies, and concerns exist regarding its clinical relevance [7-9], as it is hard to induce pure tensile forces intraorally [8,10]. In addition, the lack of standardization and the difficulty in interpreting and linking the in vitro findings with the actual clinical effectiveness presented challenges to the validity of this test [1,7].
New testing methodologies have been introduced to overcome those limitations, including flexural bond strength test, Brazilian bond strength test, and interfacial fracture toughness test. Flexural (bending) tests are favored to verify the strength of adhesive junctions. Since it offers a suitable model for mimicking or representing the intraoral loading environments and sustains uniform stresses at the bonded interface precluding critical errors that can influence the test findings [8,11]. Occlusal forces usually have a lever effect at the end of the restoration comparatively analogous to the stress situation created by the loading axis plane of a flexural test specimen [8]. In comparison to 3-point bending test, the four-point flexural bond strength testing should be employed for testing bonded specimens made of dissimilar materials with elastic modulus mismatch [8,11].
In the meantime, there is insufficient research concerning the use of the flexural bond strength test as a novel testing modality to measure the bond strength compared to the μTBS test. Two resin matrix ceramic blocks are used in this study as variable substrates, and different surface treatments for accomplishing bonding with composite resin; in case of intraoral repair are used as experimental variables. For the given reasons, the aim of this study was to compare the two testing methodologies (μTBS test and FBS test) regarding their modes of failure and reliability. The first null hypothesis was that the proposed surface treatments do not affect the μTBS and FBS of the bonded resin matrix ceramic. The second null hypothesis was that the type of resinmatrix ceramic material has no effect on the μTBS and FBS values. The third null hypothesis was that there is no difference between the validity and reliability of μTBS and FBS tests.
Materials and Methods
The materials used in this study are presented in Table 1. The sample size was calculated for the μTBS and FBS tests based on an internal pilot study data gained using G*Power version 3.1.9.2. For sample size analysis at α=0.05 and 80% power, the effect size for μTBS test was equal to 0.5202, that produces a sample size of 12 specimens per group. While the effect size for FBS test was equal to 0.4804, that yields a sample size of 13 specimens per group. Fifteen specimens were prepared for each group to gain extra power.
Material | Brand name | Composition | Manufacturer | Lot number |
---|---|---|---|---|
Polymer infiltrated ceramic | VITA Enamic | Polymeric matrix 14 wt.% (UDMA, TEGDMA) | Vita Zahnfabrik, | 55313 |
Fillers 86 wt.%: SiO2 (58–63%), Al2O3 (20–23%), Na2O (9–11%), K2O (4–6%), B2O3 & ZrO2 (<2%). CAD/CAM block size (12 x 14 x 18mm). | Bad Säckingen, Germany | |||
Resin nanoceramic | Lava Ultimate | Bis-GMA, UDMA, Bis-EMA, TEGDMA, SiO2 (20 nm), ZrO2 (4–11 nm), ZrO2/SiO2 clusters, filler mass (80 wt. %). CAD/CAM block size (12 x 14 x 18mm). | 3M ESPE, St Paul, MN, USA | N770935 |
Universal adhesive | Single bond | 10 Methacryloyloxydecyl dihydrogen phosphate, HEMA, silane, dimethacrylate resins, Vitrebond, copolymer, filler, ethanol, water, initiators | 3M ESPE, St Paul, MN, USA | 5695133 |
Nanohybrid composite resin | Brilliant NG | Methacrylates, dented glass, and amorphous silica, with filler content 80 wt.% | Coltene, Altstätten, Switzerland | 162456 |
Hydrofluoric acid etchant | Porcelain etchant | 9.5% buffered hydrofluoric acid gel | Bisco, Irving Park Rd. Schamburg, IL, USA | 2000001191 |
Table 1: Materials used in the study, their brand names, compositions, manufacturers, and lot numbers.
The prefabricated Vita Enamic (VE)and Lava Ultimate (LU) blocks were cut using a low-speed precision cutting saw (Isomet 4000, Buehler, Lake Bluff, IL, USA) with a 7-inch low-concentration diamond wafer blade (Kemet, Maidstone, UK), into smaller blocks (5 x 12 x 14mm). The cutting procedure was performed under profuse water coolant at 3200 rpm and a 6 mm/min feed rate.
The cut blocks were wet-polished in a unidirectional circular motion for 1 minute with silicon carbide papers of 600, 800, 1000, and 1200 grit, respectively, to standardize the surface roughness. The polished blocks were ultrasonically cleaned in distilled water for 5 minutes [12]. Afterward, each type of RMC block was allocated into four subgroups according to the following surface treatments.
Diamond bur grinding group (DBR): the polished surface was ground using a standard wheel stone for five consecutive strokes in the same direction, using a water-cooled high-speed handpiece with light pressure [13]. Diamond bur grinding + silane application group (DBR+S): The same procedure as DBR followed by air-drying of the surface and the application of silane coupling agent for 60 seconds, then 5 seconds of gentle drying [14]. Hydrofluoric acid etching group (HF): the polished surface was etched with 9.5% HF acid for 60 seconds and rinsed for 60 seconds using an air-water spray [15]. Hydrofluoric acid etching+ saline application group (HF+S): The same as the HF acid etching group after the surface was dried, the silane coupling agent was applied for 60 seconds, and gently dried for 5 seconds [14]. Following the surface treatments, the single bond universal adhesive was applied according to the manufacturer’s instructions. It was actively applied on the treated surface for 20 seconds and softly air-dried with air-water spray until no movement of the adhesive layer was observed, indicating solvent evaporation. Then, the adhesive was light- cured for 10 seconds in four overlapping cycles to polymerize the whole treated surface.
The nanohybrid composite was built up on the surfacetreated blocks in increments of 2 mm thickness and lightcured for 40 seconds in four overlapping cycles to ensure complete polymerization of the composite layer using an LED light-curing unit (Elipar, S10, 3M ESPE, Germany) of output intensity 1200 mW/cm2. Incremental packing of composite continued until the composite build-up of (5 mm height x 12mm width x 14 mm length) was achieved [14]. The bonded blocks were stored in an incubator (Titanox, art.a3-213-400I, Italy) at 37 degrees Celsius for 24 hours in distilled water in a dark container to ensure post-curing polymerization [16]. All bonded blocks were cut longitudinally perpendicular to the bonded interface in the X-axis and Y-axis directions [7,17], using the Isomet 4000 to produce beam-shaped specimens (0.9 x 0.9 x 10 mm). A total count of 240 beam-shaped specimens were obtained and were randomly divided into 2 groups according to the type of bond strength test (n=15).
Microtensile bond strength (μTBS) testing
The beams were aligned on the testing jigs so that the bonded interface is perpendicular to the loading axis and fixed in this position by gluing the beam’s edges to the upper and lower parts of the special testing jig using cyanoacrylate glue. The specimens were subjected to tensile forces in the universal testing machine (Instron 3356, UK) at a crosshead speed of 0.5 mm/min till fracture [7,18]. μTBS values in MPa were determined automatically by the Bluehill 3 software using this equation (Maximum load at fracture in N/surface area in mm2).
Flexural Bond strength (FBS) testing
The beams were tested in a four-point bending setup, with two rounded loading points 1.8 mm apart and two rounded supporting points 7.2 mm apart. The beams were placed so that the bonded interface was centralized between the loading points using a transparent grid. The specimens were tested by using a universal testing machine (Instron 3365) with a crosshead speed of 0.5 mm/min till fracture [7] (Figure 1). The flexural bond strength was calculated using a customized equation made by Bluehill software 3.
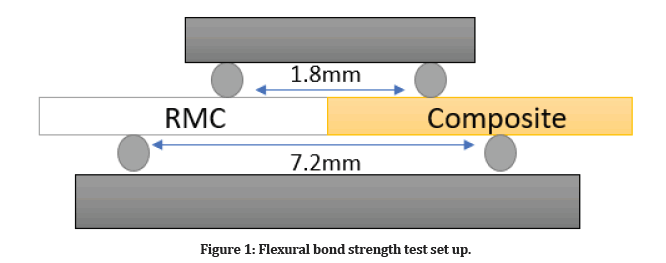
Figure 1: Flexural bond strength test set up.
Failure mode analysis
After the μTBS and FBS tests, the fractured beams’ modes of failure were assessed using a stereomicroscope [19] (Olympus DP10, Olympus optical co. LTD) with a magnification of 40x. The failure modes were categorized into three categories: adhesive failure at the bonded interface, cohesive failure in either of the substrates and mixed failure comprising both adhesive and cohesive failure [17].
Statistical analysis
Statistical analysis was performed by using SPSS (statistical package for social sciences, IBM SPSS Statistics for Mac, version 24 software, Armonk, NY: IBM Corp, USA). The data were tested for normality using the Kolmogorov – Smirnov test and the Shapiro-test. The results were found to be normally distributed. Statistical analysis was carried out using factorial analysis of variance (ANOVA) to inspect the effect of the material and surface treatments on the microtensile and flexural bond strength. Following significant interactions, an independent t-test was performed to investigate the effect of the material and One-way ANOVA to evaluate the effect of surface treatments on the microtensile and flexural bond strength. The association between the type of test and failure modes was studied using the Chi-square test for independence. Weibull distribution parameters were estimated using the maximum likelihood method (R statistical analysis software version 4.1.0 for Windows).
Results
Numerical data were explored for normality by using the Shapiro-Wilk test and variance homogeneity by the Levene test. The data showed parametric distribution and homogeneity of variances across the groups and were presented as mean and standard deviation values. The mean and standard deviation of μTBS and FBS are shown in Table 2. The independent T-test indicated that the “Lava ultimate” groups showed higher mean bond strength values compared to the “Vita Enamic” groups (p<0.01) regardless of the effect of surface treatments. Except for the FBS value for the (DBR+S) group, there was no significant difference between the two materials. Failure modes distribution for microtensile bond strength test and flexural bond strength are illustrated in Figure 2. Weibull moduli and characteristic strength of all groups for μTBS and FBS estimated by the maximum likelihood estimation method are shown in Tables 3 and 4, respectively.
Type of test | Surface treatments | Vita Enamic (VE) | Lava Ultimate (LU) |
---|---|---|---|
µTBS test | DBR | 30.83 ± (4.04) b | 44.84 ± (4.99) bc |
DBR+S | 44.26 ± (5.55) a | 55.98 ± (5.72) a | |
HF | 26.80 ± (2.81) c | 40.07 ± (5.37) c | |
HF+S | 42.28 ± (3.40) a | 47.48 ± (4.55) b | |
FBS test | DBR | 100.07 ± (8.52) b | 151.78 ± (7.25) ab |
DBR+S | 154.45 ± (15.40) a | 155.91 ± (15.99) a | |
HF | 106.88 ± (11.83) b | 143.89 ± (8.03) b | |
HF+S | 153.52 ± (14.01) a | 143.13 ± (13.52) b | |
Different lowercase letters within the same column indicate significant differences between different surface treatments |
Table 2: Mean values and standard deviation of microtensile bond strength (µTBS) test (MPa) and flexural bond strength (FBS) test (MPa).
Material | Surface treatments | Weibull modulus (m) | 95% confidence interval for m | Characteristic strength (CI) (MPa) | 95% confidence interval for CI | ||
---|---|---|---|---|---|---|---|
Lower bound | Upper bound | Lower bound | Upper bound | ||||
Vita Enamic | DBR | 8.19 | 5.17 | 11.21 | 32.57 | 30.43 | 34.7 |
DBR+S | 8.8 | 5.46 | 12.15 | 46.68 | 43.83 | 49.52 | |
HF | 10.05 | 6.14 | 13.97 | 28.04 | 26.69 | 29.38 | |
HF+S | 13.16 | 8.17 | 18.15 | 43.86 | 42.06 | 45.65 | |
Lava Ultimate | DBR | 11.72 | 6.76 | 16.67 | 46.96 | 44.83 | 49.09 |
DBR+S | 10.72 | 6.54 | 14.89 | 58.45 | 55.92 | 60.99 | |
HF | 10.08 | 5.7 | 14.46 | 42.28 | 40.06 | 44.51 | |
HF+S | 12.72 | 7.5 | 17.95 | 49.44 | 47.46 | 51.42 | |
*CI: Characteristic strength at 63.2% probability of failure |
Table 3: Weibull parameters estimated by the maximum likelihood estimation method for the Microtensile bond strength test.
Material | Surface treatments | Weibull modulus (m) | 95% confidence interval for m | Characteristic strength (CI) (MPa) | 95% confidence interval for CI (MPa) | ||
---|---|---|---|---|---|---|---|
Lower bound | Upper bound | Lower bound | Upper bound | ||||
Vita Enamic | DBR | 11.62 | 104.06 | ||||
7.41 | 15.82 | 99.24 | 108.88 | ||||
DBR+S | 13.13 | 7.54 | 18.73 | 161.06 | 154.53 | 167.58 | |
HF | 11.19 | 6.7 | 15.67 | 112.15 | 106.16 | 118.14 | |
HF+S | 13.49 | 8.03 | 18.95 | 159.62 | 153.31 | 165.94 | |
Lava Ultimate | DBR | 19.77 | 12.8 | 26.75 | 155.33 | 151.1 | 159.56 |
DBR+S | 12.3 | 7.22 | 17.38 | 163.15 | 154.97 | 171.33 | |
HF | 22.51 | 13.4 | 31.62 | 147.44 | 143.95 | 150.94 | |
HF+S | 13.31 | 7.78 | 18.84 | 149.15 | 142.88 | 155.43 | |
*CI: Characteristic strength at 63.2% probability of failure |
Table 4: Weibull parameters estimated by the maximum likelihood estimation method for the Flexural bond strength test.
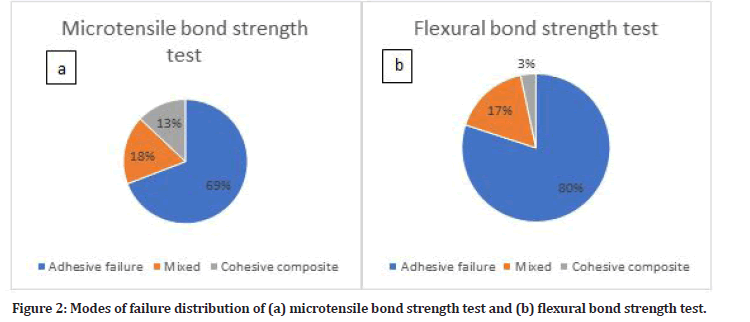
Figure 2: Modes of failure distribution of (a) microtensile bond strength test and (b) flexural bond strength test.
Discussion
The proposed null hypotheses were all rejected as there was a difference between the surface treatments and their effect on the two RMC types. There was a difference between the two RMCs on the μTBS test and FBS. Moreover, there was a difference between the μTBS test and FBS. The LU material showed higher bond strength values for both testing methodologies with all surface treatments in comparison to the VE. This could be attributed to the great mismatch in the elastic modulus of VE as it is three times the elastic modulus of LU [20]. The lower the elastic modulus, the more stress dissemination throughout the material. The high elastic modulus of VE material results in stress concentration at the bonded interface [21].
However, there was no difference in the FBS values for the group (DBR+ Silane application) between the two materials unlike the microtensile bond strength results. During the tensile test, tensile stresses generated inside the specimen can magnify the cracks leading to catastrophic failure [22]. Thus, the probability of cohesive fracture is more frequent to occur in substrates, especially those with brittle nature and inherent flaws. Cohesive failure in the cases of high bond strength values is common [22], which is in this study, the (DBR+ Silane application) surface treatment.
Conforming to the manufacturers’ descriptions, the ceramic/polymer ratio by weight for VE and LU are 86/14% and 80/20%, respectively [20]. A prior study stated that the repair bond strength was great in CAD/ CAM blocks with higher resin content [21]; these marked discrepancies in the ceramic/polymer ratios may justify the inferior flexure strength of the VE. Furthermore, LU holds 31% zirconia fillers among its inorganic structure, promoting its flexural strength [20].
In our study, the difference in the FBS and μTBS values agrees with previous studies [7, 23]. In mechanical testing, the flexural strength is substantially higher than the tensile strength. This difference could be accredited to the size effect; because the area of the interface subjected to tensile stresses in the four-point bending test is small compared to the interface of the microtensile test in which the tensile stresses are uniformly distributed across the bonded interface [22]. The modes of failure distribution reveal; that most FBS test specimens experienced adhesive failure, whereas a mixture failure mode occurred for the μTBS test. This finding agrees with previous studies [7, 9]. Postulating that, the FBS test is probably a reliable test method for bond assessment since it has been proposed that adhesive failures entail the ‘true’ adhesive strength [9]. Weibull statistics were implicated to assess the validity of FBS test in comparison to μTBS test. The Weibull modulus is the slope of the Weibull plot, and it depends on the flaw distribution in the test specimen. The higher the Weibull modulus, the more consistent and reliable the testing results. The Weibull modulus is not a material constant; because it differs according to cracks distribution, distances, and interactions between cracks geometry and stress areas [23]. In the current study, the only difference between the two tests was in the test mechanics. Since the materials used, surface treatments applied, preparation methodology, cutting procedure, and crosshead speeds during testing were the same. Thus, the difference in the Weibull moduli between the two tests accounts for the difference in the test mechanics, sensitivity, and reliability. In this study, the Weibull module for FBS were generally higher than the μTBS, and this was consistent with the results of a previous study [15]. In addition, another study that compared the two tests using larger specimen dimensions with greater probability of flaws showed similar results to the present study [9].
The low Weibull modulus for the μTBS test indicates the greater diversity in the results. This large variability could be due to the difficulty of mounting the specimens on the jigs, as it could easily slip from the glue due to its smooth surface. Furthermore, during gluing the specimens, any minute change in alignment or slight tilt can cause shear stresses at the adhesive interface affecting the result [9]. Moreover, pretest failures commonly occurred while mounting the specimens, and the whole equipment setup reflects the high sensitivity of the μTBS test [9]. On the other hand, the FBS test seemed more reliable, reproducible, and easy to perform.
Conclusion
Based on the findings of this in vitro study, it could be concluded that:
Flexural bond strength test (4-point bending test) could be employed as a reliable, unsophisticated alternative approach to μTBS test to assess the bond strength for dental applications.
References
- El Mourad AM. Assessment of bonding effectiveness of adhesive materials to tooth structure using bond strength test methods: A review of literature. Open Dent J 2018; 12:664-78.
- De Munck J, Luehrs AK, Poitevin A, et al. Fracture toughness versus micro-tensile bond strength testing of adhesive-dentin interfaces. Dent Mater 2013; 29:635-644.
- Armstrong S, Breschi L, Ozcan M, et al. Academy of dental materials guidance on in vitro testing of dental composite bonding effectiveness to dentin/enamel using micro-tensile bond strength (muTBS) approach. Dent Mater 2017; 33:133-43.
- Van Meerbeek B, Peumans M, Poitevin A, et al. Relationship between bond-strength tests and clinical outcomes. Dent Mater 2010; 26:e100-121.
- Roeder L, Pereira PN, Yamamoto T, et al. Spotlight on bond strength testing--unraveling the complexities. Dental Mater 2011; 27:1197-203.
- Neves Ade A, Coutinho E, Cardoso MV, et al. Influence of notch geometry and interface on stress concentration and distribution in micro-tensile bond strength specimens. J Dent 2008; 36:808-815.
- El Gezawi M, Haridy R, Abo Elazm E, et al. Microtensile bond strength, 4-point bending and nanoleakage of resin-dentin interfaces: Effects of two matrix metalloproteinase inhibitors. J Mech Behav Biomed Mater 2018; 78:206-213.
- Sanli S, Comlekoglu MD, Comlekoglu E, et al. Influence of surface treatment on the resin-bonding of zirconia. Dent Mater 2015; 31:657.
- Wong ACH, Tian T, Tsoi JKH, et al. Aspects of adhesion tests on resin-glass ceramic bonding. Dent Mater 2017; 33:1045-1055.
- Akay C, Tanis MC, Mumcu E, et al. Influence of nano alumina coating on the flexural bond strength between zirconia and resin cement. J Adv Prosthodont 2018; 10:43-49.
- Komurcuoglu MB, Sagirkaya E, Tulga A. Influence of different surface treatments on bond strength of novel CAD/CAM restorative materials to resin cement. J Adv Prosthodont 2017; 9:439-446.
- Celik E, Sahin SC, Dede DO. Effect of surface treatments on the bond strength of indirect resin composite to resin matrix ceramics. J Adv Prosthodont 2019; 11:223-231.
- Zaghloul H, Elkassas DW, Haridy MF. Effect of incorporation of silane in the bonding agent on the repair potential of machinable esthetic blocks. Eur J Dent 2014; 8:44-52.
- Bello YD, Di Domenico MB, Magro LD, et al. Bond strength between composite repair and polymer-infiltrated ceramic-network material: Effect of different surface treatments. J Esthet Restor Dent 2019; 31:275-279.
- Lise DP, Van Ende A, De Munck J, et al. Microtensile bond strength of composite cement to novel CAD/CAM materials as a function of surface treatment and aging. Operative Dent 2017; 42:73-81.
- Rand Mohammed Falah ZMAA. Shear bond strength of aged CAD/CAM ceramic materials repaired by resin composite in vitro using different repair adhesives. J Res Med Dent Sci 2020; 8:61-67.
- Zahra Khamverdi SK, Nafiseh Fazelian, Mahdi Akbarzadeh. Effect of tubular orientation on the microtensile bond strength of composite-dentin using universal bonding agents. J Res Med Dent Sci 2018; 6:8.
- Morad Sadaghiani MR, Keyvan Saati, Hadi Kokabi, et al. Comparison of the micro-tensile bond strength of composite resin restoration in micro- and nano-hybrid composite resins using different interfacial materials. J Res Med Dent Sci 2018; 6:436-44.
- Kaimal A, Ramdev P, Shruthi CS. Evaluation of effect of zirconia surface treatment, using plasma of argon and silane, on the shear bond strength of two composite resin cements. J Clin Diagn Res 2017; 11:ZC39.
- Sismanoglu S, Tugce Gurcan A, Yildirim‐Bilmez Z, et al. Mechanical properties and repair bond strength of polymer‐based CAD/CAM restorative materials. Int J Appl Ceram Technol 2020; 18:312-318.
- Bayraktar Y, Demirtağ Z, Çelik Ç. Effect of Er:YAG laser pulse duration on repair bond strength of resin-based and hybrid CAD/CAM restorative materials. J Adhes Sci Technol 2021; 36:1-14.
- Li J, Li H, Yun X, et al. A comparison of bond strengths measured using cantilever bending and micro-tensile methods. Dent Mater 2011; 27:1246-1251.
- Shin Y, Kim Y, Cho BH. Flexural test as an alternative to tensile test for bond strength of resin cement to zirconia. J Mech Beh Biomed Mater 2021; 119:104525.
Indexed at, Google Scholar, Cross Ref
Indexed at, Google Scholar, Cross Ref
Indexed at, Google Scholar, Cross Ref
Indexed at, Google Scholar, Cross Ref
Indexed at, Google Scholar, Cross Ref
Indexed at, Google Scholar, Cross Ref
Indexed at, Google Scholar, Cross Ref
Indexed at, Google Scholar, Cross Ref
Indexed at, Google Scholar, Cross Ref
Indexed at, Google Scholar, Cross Ref
Indexed at, Google Scholar, Cross Ref
Indexed at, Google Scholar, Cross Ref
Indexed at, Google Scholar, Cross Ref
Indexed at, Google Scholar, Cross Ref
Indexed at, Google Scholar, Cross Ref
Indexed at, Google Scholar, Cross Ref
Author Info
Annan Ahmed Elkassaby1*, Mohamed M Kandil1,2 and Ghada Atef Alian1
1Department of Dental Biomaterials, Dentistry, Ain Shams University, Egypt2Department of Dental Biomaterials, Galala University, Egypt
Citation: Annan Ahmed Elkassaby, Mohamed M Kandil, Ghada Atef Alian, Microtensile vs. Flexural Bond Strength for Bond Strength Assessment, J Res Med Dent Sci, 2022, 10 (8):53-58.
Received: 27-Jul-2022, Manuscript No. 67821; , Pre QC No. jrmds-22-67821(PQ); Editor assigned: 29-Jul-2022, Pre QC No. jrmds-22-67821(PQ); Reviewed: 12-Aug-2022, QC No. jrmds-22-67821; Revised: 17-Aug-2022, Manuscript No. jrmds-22-67821(R); Published: 24-Aug-2022