Research - (2020) Volume 8, Issue 4
Head and Neck Cancer Radiotherapy Effect on Some Properties of Different Dental Restorative Materials: An in Vitro Study
Sara said Eltohamy*, Mohamed M Kandil and Dina A El Refai
*Correspondence: Sara said Eltohamy, Department of Biomaterials, Ain- Shams University, Organization of African unity St, Egypt, Email:
Abstract
Aim: evaluation of the effect of HNC radiotherapy on diametral tensile strength, wear resistance, and color stability of Nanocomposite (Filtek Z-350XT), RMGI (Fuji II LC), Compomer (Composan glass).
Methods: A total of 300 specimens were prepared, 100 for each restorative material. 40 specimens from each material will not be exposed to radiation (control group). 30 specimens will be irradiated to 50 Gy and 30 specimens will be irradiated to 70 Gy. Diametral tensile strength (DTS), wear resistance, and color stability tests were measured for each group (n=10). One-way ANOVA was used to explore significance between groups followed by Tukey’s post hoc test. Independent t-test was carried to compare the color change of 50 Gy and 70 Gy groups.
Results: Reduction in DTS, and wear resistance was observed in the three materials after radiotherapy. Nano composite showed the highest DTS, and wear resistance, followed by compomer, while RMGI had the lowest values before and after radiation. Color change was observed also. RMGI had the least ΔE values, followed by nanocomposite, while compomer showed the highest ΔE values. These alterations in properties were radiation dose dependent.
Significance: The ionizing radiation doses used in HNC radiotherapy have a deteriorating effect on some properties of the three restorative materials used. Nanocomposite is the dental restorative material of choice in HNC patients undergoing radiotherapy due to its high mechanical properties and good color stability before and after radiation.
Keywords
Radiotherapy, HNC, Gamma radiation, Nanocomposite, Rmgi, Compomer
Introduction
Head and neck cancers (HNC) represent the sixth most common cancer worldwide with approximately 630,000 new patients diagnosed annually [1]. Radiotherapy is currently a widely used and important part of treatment of HNC. It can be used as a sole treatment or associated with surgery and/or chemotherapy [2]. It semi selectively destroys cancer cells while preserving normal cells [3]. It can be used as a curative, adjuvant, neo-adjuvant, and palliative type of treatment, and is often used in conservative approaches [4]. Head and neck radiotherapy consist of cumulative doses that are mostly fractionated and delivered in daily sessions with pauses on weekends. The treatment usually lasts for about 7 weeks and its total doses range from 40 to 70 Gy depending on several factors as tissue response [5].
However, Ionizing radiation has numerous adverse reactions which is more evident in the head and neck region where a variety of highly radiosensitive structures are found [6]. These complications may be xerostomia, osteoradionecrosis, and radiation caries, etc. [7]. This necessitates the need for special dental care for HNC patients subjected to head and neck radiotherapy, and the use of high performance and stable dental restorative materials. Physicians often recommend dental treatment to patients just before head and neck radiotherapy. Such treatment usually requires replacement of metal-based restorations such as amalgam with polymer-based restorative materials to avoid interaction of ionizing radiation with metallic materials, intensifying the radiation in the surroundings of the material. This secondary irradiation effect should be reduced in polymerbased materials since they absorb radiation [8]. Resin-based dental restorations such as dental composites, resin modified glass ionomers, and compomers are polymeric tooth-colored restorative materials that are widely used in many dental applications due to their good clinical performance.
In this study, the assessment of some physical and mechanical properties of three different dental restorative materials; before and after exposure to the HNC radiotherapy therapeutic dose; could show its effect on these materials, and thus allow the clinicians to choose the best material in such cases.
Materials and Methods
Materials used in the study
Restorative materials used in the study, their composition, manufacturers, and lot number are shown in Table 1.
Material | Trade name | Main constituents | Shade | Manufacturer | Lot no.# |
---|---|---|---|---|---|
Nanocomposite | Filtek Z-350XT | Nanofilled resin-based composite: resin matrix (bis-GMA, UDMA, bis-EMA, TEGDMA and PEGDMA) and 78 wt% (or 59 vol %) of zirconia/silica particles and non-agglomerated silica particles. | A3 | 3M ESPE, Dental Products, St. Paul, MN, USA |
N914048 |
RMGI | Fuji II LC | Distilled water, polyacrylic acid, HEMA, UDMA, silicon dioxide, aluminosilicate glass |
A3 | GC Corporation, Cho, Itabashi Ku, Tokyo, Japan |
180528A |
Compomer | Composan glass | BIS-GMA, TEGDMA, BHT, Diurethane-dimethacrylate |
A3 | Promedica Dental Material GmbH Domagkstrasse Neumuenster Germany |
1814118 |
Table 1: Restorative materials used, trade names, main constituents, shade, manufacturer, and lot numbers.
Specimens preparation and material testing
Split Teflon molds were used to prepare the specimens with different dimensions according to the corresponding test as follows
Diametral tensile test: 6 mm diameter x 3 mm thickness
Wear resistance test: 6mm diameter x 2mm thickness.
Color stability test: 10 mm diameter x 2 mm thickness.
First a celluloid matrix was placed over a glass slide then the mold was placed over it. The material was then condensed in a single increment and covered with a celluloid matrix and another glass slide. To obtain a flat surface free of voids, a 500-gm weight was placed over the glass slide for 1 minute with a standardized pressure. After the removal of the weight, the specimen was light cured through the glass slide according to the manufacturer’s instructions for 20 seconds using a LED light cure (3M ESPE, Elipar, Deep cure-L Germany) of 1200 mW/cm2 output, which was periodically checked every five curing sessions using a radiometer (Model 100 curing radiometer, Kerr, USA).
After mold disassembly Figure 1, specimens were stored in distilled water in an incubator at 37℃ for 24 hours before irradiation and testing.
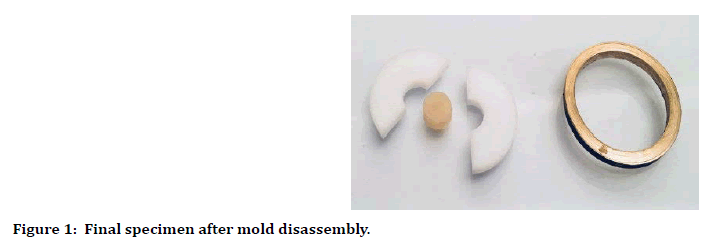
Figure 1. Surgical training questionnaire
Exposure to gamma radiation
Specimens of each material were divided into 3 groups
Specimens that were taken as control group (non-irradiated) (0 Gy).
Specimens that were exposed to radiation dose of (50 Gy).
Specimens that were exposed to radiation dose of (70 Gy).
The specimens were embedded in special phantom prepared of pink modeling wax with 1 cm thickness (tissue equivalent material), then irradiated with gamma rays in single shots [9].
The irradiation was performed at National Centre of Radiation Research and Technology, Cairo, Egypt, using 137 Cesium Gamma Cell 40 giving a dose rate of 0.658 rad/sec at the time of experiment.
Diametral tensile strength test
Specimens were individually mounted on a universal testing machine (Model 3345; Instron Industrial Products, USA) with a load cell of 5000 N. Specimens were then statically loaded diametrically using a load applicator (stainlesssteel rod with a flat end) attached to the upper movable compartment of the machine. Loading was applied at a crosshead speed of 0.5 mm/min until failure. Then the maximum failure load was recorded.
The diametral tensile strength (DTS) was calculated using the following formula
DTS=2F/πdh
Where: “F” is the fracture load (in N)
“π”=3.1416
“d” is the specimen diameter (in mm)
“h” is the specimen height (in mm) [10].
Wear resistance
The two-body wear testing was performed using a ROBOTA chewing simulator (Model ACH-09075DC-T, AD-Tech Technology Co., Ltd., Germany), which has four chambers simulating the direct contact movements of human teeth simultaneously (Figure 2). Each of the chambers consists of an upper Jackob’s chuckas tooth antagonist holder and a lower plastic specimen holder. The specimens were embedded in Teflon housing in the lower specimen holder. A weight of 5 kg, comparable to 49 N of chewing force was applied. The number of cycles for each specimen was 20,000 as per standard procedure.
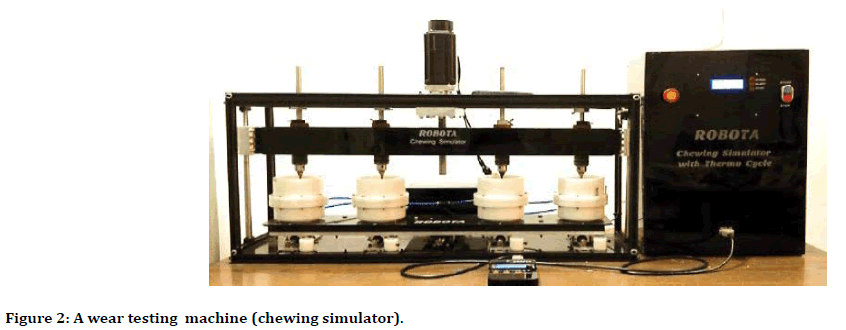
Figure 2. Surgical training questionnaire
Wear was determined from weight loss of the specimens measured by an electronic balance (with an accuracy of 0.0001 gram) according to the following formula:
Weight loss=Final weight–Original weight
Where:
Final weight=weight of the specimen after the wear test,
Original weight=weight of the specimen before the wear test
Before weighing, each mounted specimen was cleaned and dried with tissue paper. To ensure accuracy, the balance was kept at all times on a free-standing table to avoid vibrations. Also, weighing the specimens was done with the glass doors of the balance kept closed to avoid the effect of air drafts.
These measurements were done for each specimen group (0 Gy - 50 Gy - 70 Gy).
Color stability
A total of 60 specimens were prepared for the color testing: 20 specimens of each material. Specimens of each material were then divided into 2 groups (50 Gy, and 70 Gy) with 10 specimens in each group.
The baseline color measurements for each group were done before exposure to gamma radiation using a portable reflective spectrophotometer (X-Rite, model RM200QC, Neu-Isenburg, Germany). For each specimen, the bottom surface was marked so that measurements were done on the top surface. Before each measurement session the spectrophotometer was calibrated according to the manufacturer recommendations.
The aperture size was set to 4 mm and the specimens were exactly aligned with the device. A white background was used, and measurements were made according to the CIE L*a*b* color system relative to the CIE standard illuminant (D65).
These measurements were repeated for each group after exposure to gamma radiation, and then the change in color (ΔE) of the specimens was measured using the following formula:
ΔE*=([L0*−Lf*]2+[a0*−af*]2+[b0*−bf*] 2)½
Where ΔE*=color change, L*=luminance reflectance, a*=red–green color coordinate, b*=yellow-blue color coordinate, 0=baseline, f=after irradiation [11].
Statistical analysis
Numerical data were explored for normality by checking the data distribution using Kolmogorov- Smirnov and Shapiro-Wilk tests. Data showed parametric distribution so; it was represented by mean and standard deviation (SD) values. Oneway ANOVA was used to explore significance between groups followed by Tukey’s post hoc test. Independent t-test was carried to compare the color change of 50 Gy and 70 Gy groups. The significance level was set at P ≤ 0.05 within all tests.
Results
DTS results
For the three tested materials (Nano-filled composite, Compomer, and RMGI ) control specimens (0 Gy) had the highest DTS mean value followed by specimens exposed to (50 Gy) while specimens exposed to (70 Gy) had the lowest mean value.
Mean and standard deviation (SD) values of DTS (MPa) for different materials and radiation doses were presented in Table (2) and Figure (3).
Material | Radiation doses (mean ± SD) | P-value | ||
---|---|---|---|---|
0 Gy | 50 Gy | 70 Gy | ||
Nano-filled composite | 56.31 ± 5.81Aa | 47.53 ± 5.48Ba | 39.58 ± 6.39Ca | <0.001* |
Compomer | 47.16 ± 5.22Ab | 37.78 ± 4.68Bb | 34.88 ± 3.98Ba | <0.001* |
RMGI | 28.46 ± 3.30Ac | 24.10 ± 4.70ABc | 22.88 ± 3.82Bb | 0.033* |
P-value | 0.001* | 0.001* | 0.001* |
Table 2: Mean ± standard deviation (SD) of DTS (MPa) for different materials and radiation doses.
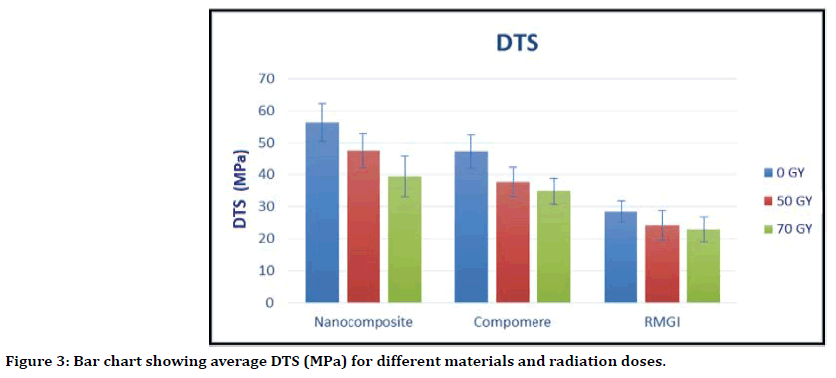
Figure 3. Surgical training questionnaire
Uppercase superscript letters (A, B, C) indicate a statistically significant difference within the same horizontal row. While lowercase superscript letters (a,b,c) indicate a statistically significant difference within the same vertical column.
Significant (p ≤ 0.05); non-significant (p>0.05).
Wear results
For the three tested materials (Nano-filled composite, Compomer, and RMGI ), specimens exposed to (70 Gy) had the highest weight loss mean value followed by specimens exposed to (50 Gy) while control specimens (0 Gy) had the lowest mean value. Mean and standard deviation (SD) values of wear measured by weight loss (gm) for different materials and radiation doses were presented in Table 3 and Figure 4.
Material | Radiation doses (mean±SD) | P-value | ||
---|---|---|---|---|
0 Gy | 50 Gy | 70 Gy | ||
Nano-filled composite | 1.33±1.00Bb | 4.78±2.82Ab | 5.33± 1.80Ab | 0.005* |
Compomer | 2.67±2.50Bab | 6.78±1.64Aab | 7.44± 1.01Aab | 0.001* |
RMGI | 5.22±3.19Ba | 8.22±4.06Aa | 9.44± 4.10Aa | 0.005* |
P-value | 0.011* | 0.030* | 0.008* |
Table 3: Mean ± standard deviation (SD) of wear measured by weight loss (gm) for different materials and radiation doses.
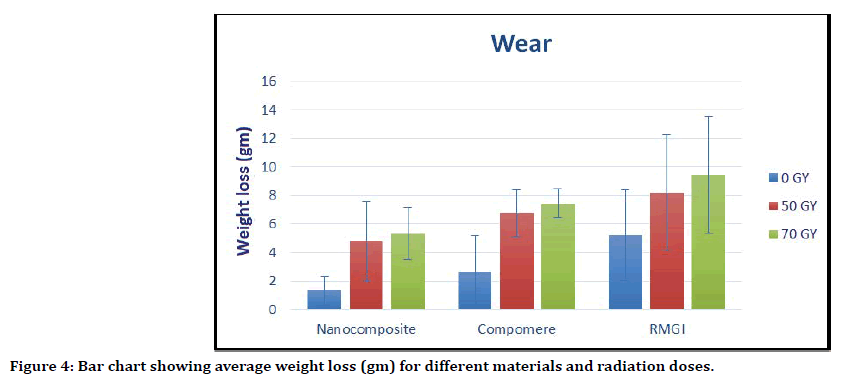
Figure 4. Surgical training questionnaire
Uppercase superscript letters (A, B, C) indicate a statistically significant difference within the same horizontal row. While lowercase superscript letters (a, b, c) indicate a statistically significant difference within the same vertical column.
Significant (p ≤ 0.05); non-significant (p>0.05).
Color stability results
Independent t-test was carried to compare the color change of 50 Gy and 70 Gy groups.
For Nano-filled composite, at the dose of (70 Gy) the color change (ΔE) of specimens was (2.59 ± 1.82), while at the dose of (50 Gy) the color change (ΔE) of specimens was (2.33 ± 1.20). The difference in the color change (ΔE) between the two groups was not significant (P=0.613).
For Compomer, at the dose of (70 Gy) the color change (ΔE) of specimens was (3.92 ± 2.56), while at the dose of (50 Gy) the color change (ΔE) of specimens was (3.79 ± 1.73). The difference in the color change (ΔE) between the two groups was not significant (P=0.799).
For RMGI, at the dose of (70 Gy) the color change (ΔE) of specimens was (2.02 ± 0.93)), while at the dose of (50 Gy) the color change (ΔE) of specimens was (1.82 ± 0.48). The difference in the color change (ΔE) between the two groups was not significant (P=0.696).
Mean and standard deviation (SD) values of color change (ΔE) for different materials and radiation doses were presented in Table 4, and Figure 5.
Material | Radiation doses (mean ± SD) | P-value | |
---|---|---|---|
50 Gy | 70 Gy | ||
Nano-filled composite | 2.33± 1.20Ab | 2.59 ± 1.82Ab | 0.613ns |
Compomer | 3.79±1.73Aa | 3.92 ± 2.56Aa | 0.799ns |
RMGI | 1.82±0.48Ab | 2.02± 0.93Ab | 0.696ns |
P-value | 0.001* | 0.001* |
Table 4: Mean ± standard deviation (SD) of color change (ΔE) for different materials and radiation doses.
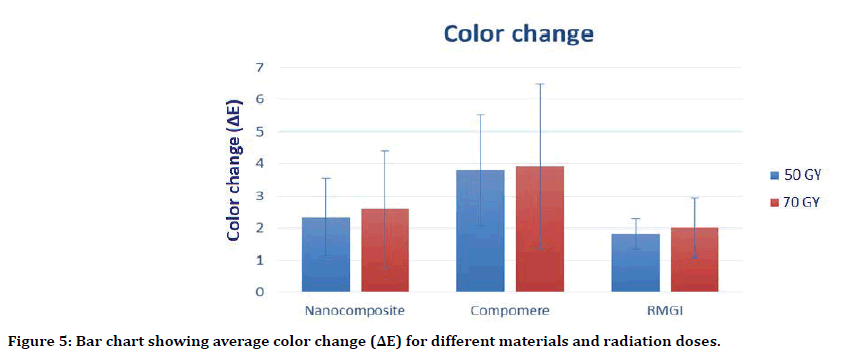
Figure 5. Surgical training questionnaire
Uppercase superscript letters (A, B, C) indicate a statistically significant difference within the same horizontal row. While lowercase superscript letters (a, b, c) indicate a statistically significant difference within the same vertical column.
Significant (p ≤ 0.05); non-significant (p>0.05).
Discussion
Radiotherapy is widely used treatment method for HNC patients. Gamma radiation therapy, a type of ionizing radiation, is one of the mostly used cancer treatments, especially for HNC patients due to its high efficiency and reliability [12]. HNC patients, suffering multiple carious lesions, could not tolerate stressful, long chairside restorative actions, so direct esthetic restorative materials could be the materials of choice for such cases.
In this study, nanocomposite, compomer, and RMGI were used as three different types of direct esthetic restorative materials due to their superior esthetics, good physical and mechanical properties, and their time saving technique which meets HNC patients' needs.
To simulate the effects of irradiation on restorative materials, a Cs-137 device was used as a source of gamma rays which is also used clinically in cancer treating radiotherapy devices. The specimens were exposed to 50 Gy and 70 Gy radiation doses, which are corresponding to the doses clinically used in patients undergoing HNC radiotherapy [13].
To study the changes occurring in the restorative materials by radiotherapy, we made testing to some of their physical and mechanical properties which are relevant to serviceability such as diametral tensile strength, wear resistance, and color stability before and after radiation.
The results of the present study showed that HNC radiotherapy protocol affects the tested properties of the three used materials as follows:
Regarding diametral tensile test, when analyzing the results of the present study, it was evident that for the three different materials, there was a significant decrease of DTS with increasing the doses of radiation. Control specimens (0 Gy) had the highest mean value followed by specimens exposed to (50 Gy) while specimens exposed to (70 Gy) had the lowest value.
Properties of the direct esthetic restorative materials may be affected by ionizing radiation such as gamma radiation. As gamma radiation has short wavelengths and high photon energy, it is believed that changes in the chemical, mechanical and physical properties of these materials can occur upon exposure to these forms of radiation [14].
The chemical alterations in the molecular structure of polymeric materials irradiated with high energy ionizing radiation has been proved in the previous studies [14]. The most obvious changes are either cross-linking of polymer chains with an increase in molecular weight or chain scission with a decrease in molecular weight and, thus, substantially changing the properties of the polymeric materials [15].
During irradiation of polymers, both chain scission and cross-linking take place, but one of these phenomena may be the most dominant depending on several factors, such as the morphology of the polymer and the irradiation environment [16].
In chain scission the breaking of C-C bonds of polymer chains by high energy radiation has been observed; with subsequently decrease in the molecular weight and the mechanical properties [17].
In our results, the three different materials behaved in the same manner. The ionizing radiation significantly decreased the DTS. This could be attributed to the presence of a polymer content in the three materials which is proved to be affected by ionizing radiation in many previous studies [18]. Thus ionizing radiation may promote the degradation and breaking of bond chains of the polymeric content of the three restorative materials decreasing significantly their DTS. This goes with previous studies which stated that mechanical properties of esthetic dental restorative materials may decrease due to the radiation effect [19].
This was in accordance with Reichmanis et al who stated that high energy ionizing radiation causes excitation and ionization in polymers, creating ions and free radicals, which likely causes polymeric chains to rupture resulting in changes in physical and mechanical
As the induced effect by the ionizing radiation is cumulative, the damage mechanisms and the rate of damage accumulation would probably be dose related [20]. This means that the higher the radiation dose, the more effective it should be. In the few studies that exist, there was a linearity between the increase of the radiation dose and its effect on dental materials mechanical properties [8]. This goes with the results of our study in which the effect of ionizing radiation on these materials increased with the increase of the radiation dose.
Regarding wear, for the three materials, the weight loss values were increased significantly by increasing the gamma radiation dose. Specimens exposed to (70 Gy) had the highest mean value followed by specimens exposed to (50 Gy) while control specimens (0 Gy) had the lowest value. These findings suggest that higher ionizing doses may promote degradation phenomenon occurred by scission of C-C bonds within the polymer content of the materials used which, in turn, could result in increased wear [19].
Regarding color stability, for the three materials, it has been found that ionizing radiation caused color change in irradiated specimens compared to control groups. These results agreed with previous studies which stated that there are color changes in the polymeric materials as a function of increasing doses of ionizing radiation [21].
These color changes can be attributed to the trapping of the excited free radicals resulting from radiation-induced rupture of polymer molecules, which have electrons with unpaired spin. Such species may also give optical discoloration [22].
One another potential cause is the presence of decomposition products of the stabilizer component of the materials. For instance, when phenolic stabilizers decompose by radiation, they may form by-products that can greatly discolor the material (23). The discoloration depends on the structure and concentration of the phenol transformation products [23].
However, under clinical conditions, 3.3 ΔE units has been shown to be necessary for the human eye to detect color differences [24]. i.e. the ΔE value less than 3.3 are clinically accepted, while the ΔE value greater than 3.7 indicates a poor match based on clinical observations, and the color difference between the observed subjects can be easily seen.
This means that compomer, with the least color stability (ΔE=3.79 ± 1.73), will show poor match when used in HNC patient, while nanocomposite (ΔE=2.33± 1.20) may be clinically accepted, and RMGI color change wouldn't be detectable to human eye (ΔE=1.82 ± 0.48)
Based on our results, one can suppose that the adverse effects observed in this study to the physical and mechanical properties of the used materials could have an impact on their clinical performance. So, patients undergoing HNC radiotherapy should be informed that the clinical survival of their esthetic dental restorations may decrease over time.
However, there is no ideal material, and the fact that irradiation changes some properties of the materials does not contraindicate their use.
The findings of this study are of paramount importance. but, the effect of ionizing radiation on dental materials is still not known completely, and additional researches, especially studies evaluating changes in the chemical composition, are required to help in understanding the mechanical behavior of materials, and to explain how the ionizing irradiation altered the properties of these materials.
Thus, more in vitro and in vivo studies are required to suggest the best dental protocols and restorative materials for patients subjected to radiotherapy, resulting in improved quality of life for these individuals [19].
The Limitation of this Study
It must be highlighted that the results of this “in vitro” study are not exactly applicable to radiation effects on dental materials in the oral environment "in vivo" where different factors may influence the restorative materials in a more complex manner than the experimental ones used in this study. Important clinical variables associated to patient biology such as xerostomia, qualitative changes in saliva, caries recurrences, and dietary changes were not evaluated. These factors can cause aging of restorations by changing their chemical and mechanical behavior.
Conclusions
Within the limitations of the current study, we can conclude that:
HNC radiotherapy had altered the physical and mechanical properties of the three used restorative materials, and these alterations were radiation dose dependent. Although exactly how this occurs has not been a studied element of this work.
Reduction in DTS, and wear resistance was observed in the three materials because of ionizing radiation.
Nano composite showed highest DTS, and wear resistance, followed by compomer, while RMGI was the lowest before and after radiation.
Color change in the three materials was observed after exposure to radiation. RMGI was clinically accepted, nano composite may be clinically accepted, while compomer was clinically unaccepted.
Funding
This research did not receive any specific grant from funding agencies in the public, commercial, or not-for-profit sectors.
References
- Parkin DM, Bray F, Ferlay J, et al. Global cancer statistics, 2002. Cancer J Clinicians 2005; 55:74-108.
- Devi S, Singh N. Dental care during and after radiotherapy in head and neck cancer. Natl J Maxillofac Surg 2014; 5:117-125.
- Chen HHW, Kuo MT. Improving radiotherapy in cancer treatment: Promises and challenges. Oncotarget. 2017; 8:62742-62758.
- Marta GN, Silva V, de Andrade Carvalho H, et al. Intensity-modulated radiation therapy for head and neck cancer: systematic review and meta-analysis. Radiotherapy and oncology. J European Society Therapeutic Radiol Oncol 2014; 110:9-15.
- Lieshout HF, Bots CP. The effect of radiotherapy on dental hard tissue: A systematic review. Clin Oral Investigations 2014; 18:17-24.
- Cardoso MDFA, Novikoff S, Tresso A, et al. Prevenção e controle das seqüelas bucais em pacientes irradiados por tumores de cabeça e pescoço. Radiologia Brasileira 2005; 38:107-115.
- De Sanctis V, Bossi P, Sanguineti G, et al. Mucositis in head and neck cancer patients treated with radiotherapy and systemic therapies: Literature review and consensus statements. Critical Reviews Oncol Hematol 2016; 100:147-166.
- Cruz AD, Sinhoreti MAC, Ambrosano GM, et al. Effect of therapeutic dose X rays on mechanical and chemical properties of esthetic dental materials. Materials Res 2008; 11:313-318.
- Sedky R, Amin A, Ibrahim M. Effect of radiotherapy on the microleakage of composite adhesive system. Ain Shams Dent J 2014; 17:11-18.
- Szczesio-Wlodarczyk A, Rams K, Kopacz K, et al. The influence of aging in solvents on dental cements hardness and diametral tensile strength. Materials 2019; 12:2464.
- Chakravarthy Y, Clarence S. The effect of red wine on colour stability of three different types of esthetic restorative materials: An in vitro study. J Conserv Dent 2018; 21:319-323.
- da Silva EM, Mansano ESB, Miazima ES, et al. Radiation used for head and neck cancer increases virulence in Candida tropicalis isolated from a cancer patient. BMC Infect Dis 2017; 17:783.
- Reed R, Xu C, Liu Y, et al. Radiotherapy effect on nano-mechanical properties and chemical composition of enamel and dentine. Archives Oral Biol 2015; 60:690-697.
- Viero F, Boscolo F, Demarco F, et al. Effect of radiotherapy on the hardness and surface roughness of two composite resins. Gen Dent 2011; 59:168-172.
- Reichmanis E, Frank CW, O'Donnell JH, et al. Radiation effects on polymeric materials: A brief overview. ACS Publications 1993.
- Kopal I, Vršková J, Labaj I, et al. The effect of high-energy ionizing radiation on the mechanical properties of a melamine resin, phenol-formaldehyde resin, and nitrile rubber blend. Materials 2018; 11:2405.
- Haque S, Takinami S, Watari F, et al. Radiation effects of carbon ions and gamma ray on UDMA based dental resin. Dent Materials J 2001; 20:325-338.
- Navarro R, Burillo G, Adem E, et al. Effect of ionizing radiation on the chemical structure and the physical properties of polycaprolactones of different molecular weight. Polymers 2018; 10:397.
- E Lima R, De Vasconcelos L, Pontual M, et al. Effect of ionizing radiation on the properties of restorative materials. Indian J Dent Res 2019; 30:408-413.
- Naves LZ, Novais VR, Armstrong SR, et al. Effect of gamma radiation on bonding to human enamel and dentin. Supportive care in cancer. Official J Multinational Association Supportive Care Cancer 2012; 20:2873-2878.
- Nouh SA, Bahareth RA. Effect of electron beam irradiation on the structural, thermal, and optical properties of poly (vinyl alcohol) thin film. Radiation Effects Defects Solids 2013; 168:274-285.
- Abutalib M. Effect of gamma irradiation on the structural and color properties of CR 6-2 Polycarbonate. Arab J Nuclear Sci Applications 2015; 48:53-60.
- Pospı́šil J, Habicher WD, Pilař J, et al. Discoloration of polymers by phenolic antioxidants. Polymer Degradation Stability 2002; 77:531-538.
- Chang JY, Chen WC, Huang TK, et al. Evaluation of the accuracy and limitations of three tooth-color measuring machines. J Dent Sci 2015; 10:16-20.
Author Info
Sara said Eltohamy*, Mohamed M Kandil and Dina A El Refai
Department of Biomaterials, Ain- Shams University, Organization of African unity St, El-Qobba Bridge, Al Waili, Cairo Governorate, EgyptCitation: Sara said Eltohamy, Mohamed M Kandil, Dina A El Refai, Head and Neck Cancer Radiotherapy Effect on Some Properties of Different Dental Restorative Materials: An in Vitro Study, J Res Med Dent Sci, 2020, 8 (4):153-161.
Received: 26-Jun-2020 Accepted: 23-Jul-2020